Fish communities provide important ‘signals’ about the ecosystem health of estuaries. Their component species typically occupy habitats from the top to bottom of the water column, feed across most levels of estuarine food webs, and encompass varied behavioral traits and estuarine use patterns. Taking a community-wide view can thus reveal many clues about the cumulative and complex impacts of the estuarine environment on higher ecological health. As a result, biotic indices that integrate key traits of fish communities, scored against established ‘benchmark’ or reference conditions, have been used to measure, monitor and report the ecological health of estuaries worldwide.
This study focused on applying a Fish Community Index (FCI) to the Peel-Harvey Estuary to measure and track its ecological health over a 40-year period from 1978 to 2018. It also aimed to link patterns in the FCI to key environmental drivers to support forecasting of ecological health under different environmental scenarios.
The FCI, which was developed specifically for south-western Australian estuaries, synthesises complex information from different structural and functional measures (metrics) of the fish community into simple ‘report card’ grades (A, very good, to E, very poor). The long historical fish community data set that exists for the Peel-Harvey was supplemented by two further years of fish sampling in the current study (2016-2018), which covered a greater spatial range in both the shallow (<1.5 m deep) and deeper waters (>1.5 m deep) than had ever been sampled previously. While this 40 year data set provides a rare basis for setting metric benchmark conditions (especially in the shallow basins where most historical data was collected), inconsistencies in sampling methods over time, gaps in the data record, and a relative lack of data in the deeper waters and rivers, has hampered some interpretations of longer-term health. Moving forward, we have proposed a consistent fish monitoring regime for the Peel-Harvey to enable its ecological health to be reliably tracked with the FCI into the future.
Based on the available evidence, our findings show that there has been a slight improvement in the health of the shallow river environments from 1978-2018, from poor (grade D) to fair (grade C), while the shallow basin waters have remained in fair health. The condition of the deeper waters, however, and especially in the basins, has declined from a good health status (grade B) to a fair/poor status (grade C/D). The improvements in the shallow rivers mainly reflect an increased dominance of small hardyhead species that are specialist feeders and can spawn in the estuary, rather than the opportunistic or detritus-feeding species that previously dominated these areas. The more obvious decline in the deeper basins, however, reflected a clear shift in the fish fauna from one that was relatively species-rich, abundant and contained more benthic-associated and/or estuarine individuals (all of which positively influence the FCI, e.g. Estuary cobbler, Mulloway, Yellow-finned whiting and Western striped grunter), to one that is more depauperate (with no fish being caught on several sampling occasions), sporadic and dominated by marine species (traits which negatively influence the FCI, e,g. Tailor, Southern eagle rays, Herring and Weeping toadfish). The opening of the Dawesville Cut in the mid-1990s, combined with a drying climate, has clearly led to a ‘marinisation’ of the fish fauna. However, the above declines in ecological health may also reflect (i) declines in the health of the benthic invertebrate fauna upon which many of these species feed, (ii) decreases in green macroalgal growths (especially in Peel Inlet) which can provide abundant fish food and shelter, (iii) increased water clarity and thus net avoidance and/or (iv) fishing pressure impacts on targeted species.
More detailed analysis of the current (2016–2018) FCI trends showed that, overall, the estuary is in fair to poor health (grades C–D). At a finer regional and seasonal resolution, trends in the shallow nearshore waters were often inconsistent, possibly reflecting that large areas of the basins are extremely shallow and prone to rapid environmental changes, and are dominated by small, highly-schooling species which can lead to variable catches. One worrying trend that was consistent in the shallows, however, was the often poor to very poor health (grade D–E) in the Mandurah Entrance Channel. This reflected a far lower number of species since the 1970s/80s, as well as clear drops in abundance of various specialist feeding, estuarine-spawning and/or bottom-dwelling species (e.g. Long-finned goby, Bridled goby and Small-toothed flounder), and increases in the relative proportions of opportunistic species such as Weeping toadfish.
Trends in the deeper waters were generally more discernible, and indicated that (i) the health of the basins is generally better than in the rivers (and especially in the Murray River); (ii) the more tidally-flushed basin regions (northern Harvey Estuary, western Peel Inlet and, to a lesser extent, eastern Peel Inlet) are often in better health (good to fair, grades B–C) than the poorly flushed southern Harvey (fair to poor, grades C–D), and (iii) the upper Murray River appears to be in chronically poor ecological condition (grade D), characterised in most seasons by only two or three species which are either detritivores or opportunistic omnivores (Sea mullet, Perth Herring or Black Bream). The population status of the latter species, which is prized by recreational fishers, is also vulnerable due to poor recruitment of juveniles and low abundances of adults above minimum legal length at capture. These trends most likely reflect the susceptibility of the upper Murray to salinity stratification of the water column, high organic matter loading, and the persistent low dissolved oxygen levels that develop as a result.
Finally, when FCI scores were statistically correlated with a suite of environmental variables, bottom water age, dissolved oxygen content and salinity were shown to have the greatest influence on ecological condition. In the deeper waters of the rivers, the best ecological condition occurred when dissolved oxygen content was highest and when the bottom water age was between 50 and 100 days. Poor FCI scores in this region occurred shortly after flooding (very low water age) and when bottom water became stagnant (water age >100 days). In the shallow waters of the rivers, increasing salinity had a weak positive effect on FCI scores, while in the shallow waters of the basins, hypoxia area (% of region with dissolved oxygen content < 2 mg/L) had a weak negative influence. These quantitative responses of the FCI to specific environmental variables provides a basis for predicting the future ecological condition of the system under various environmental scenarios.
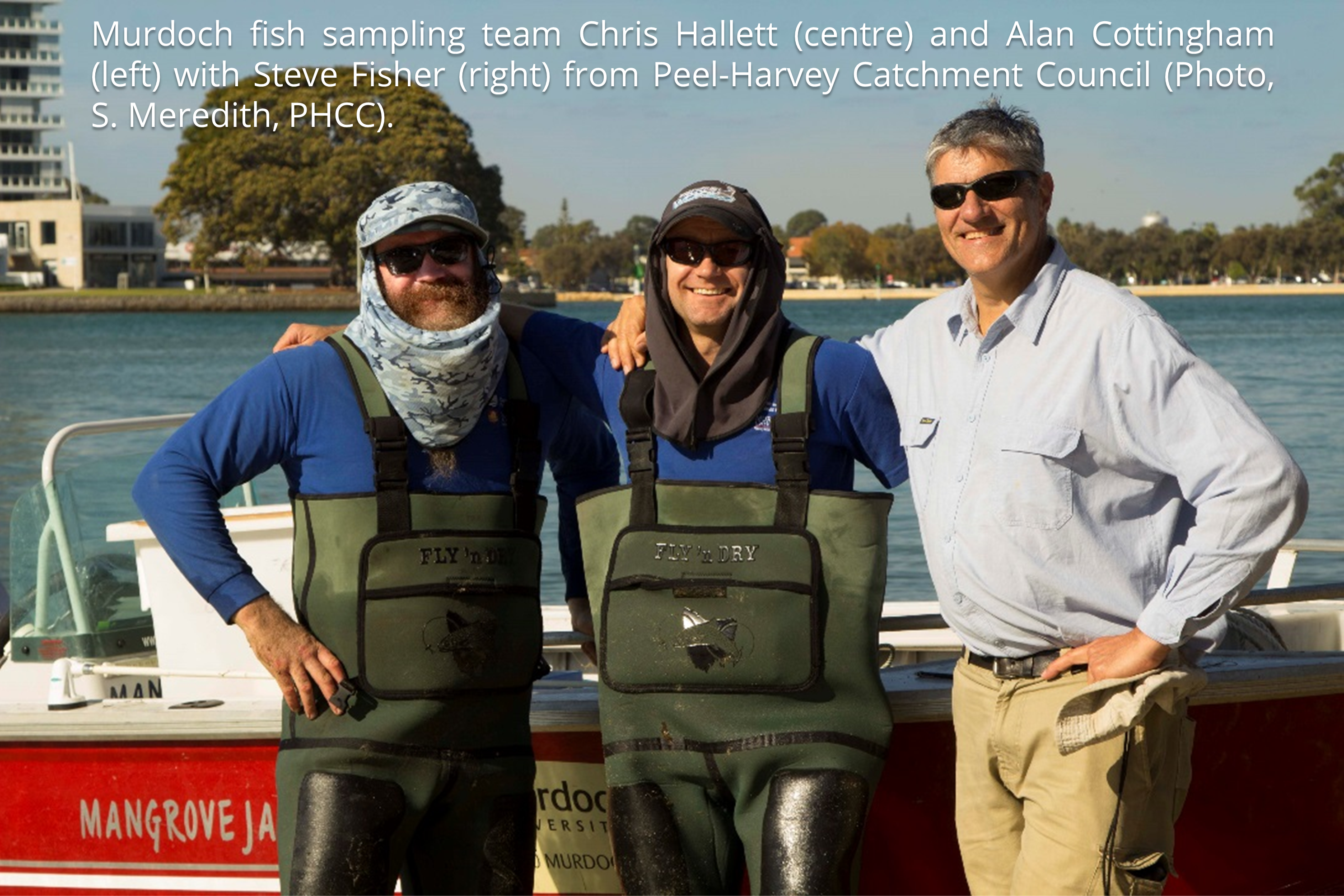